The quest to save the banana from extinction
You might never be quite sure what to expect when you peeled a banana
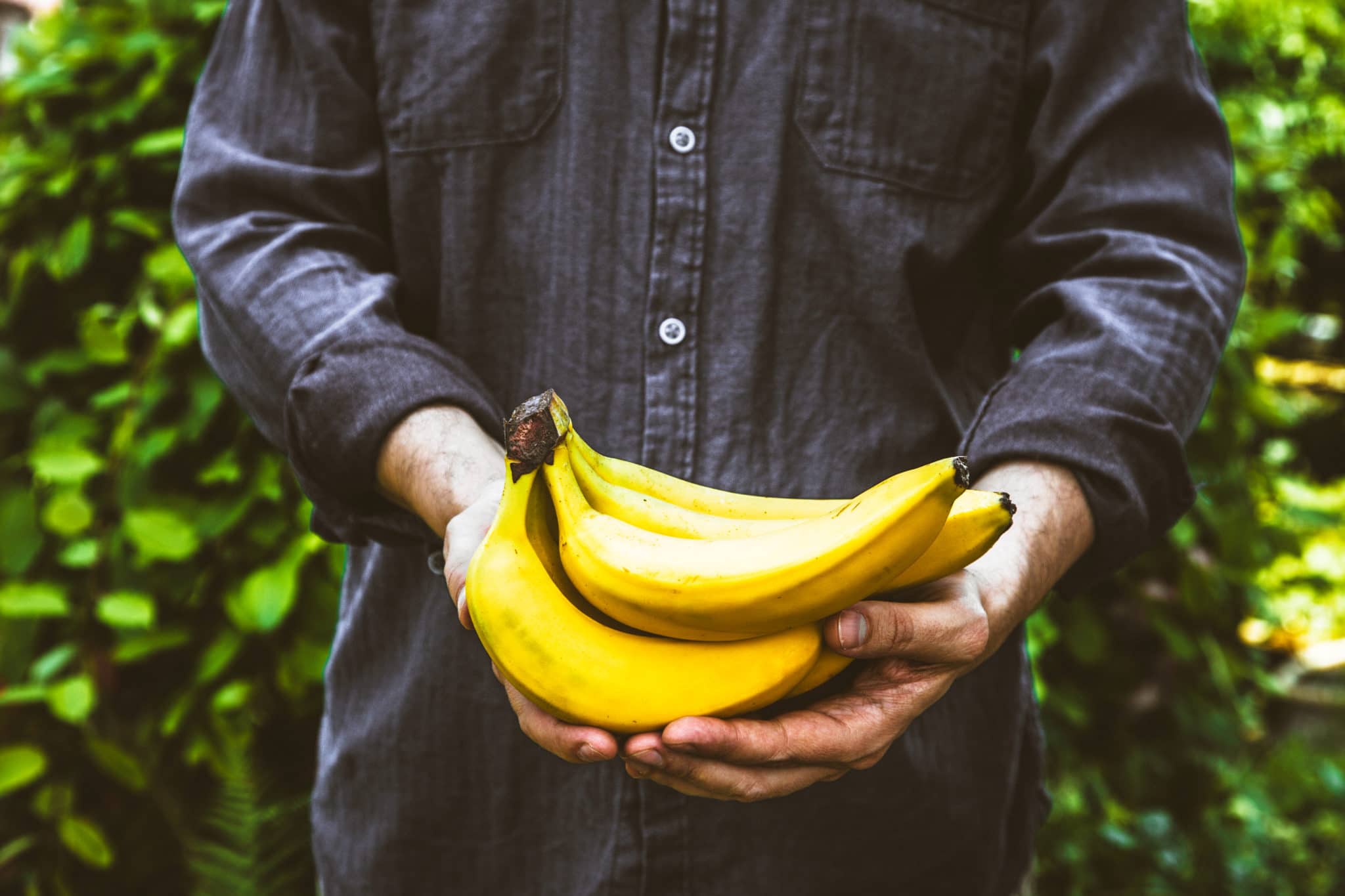
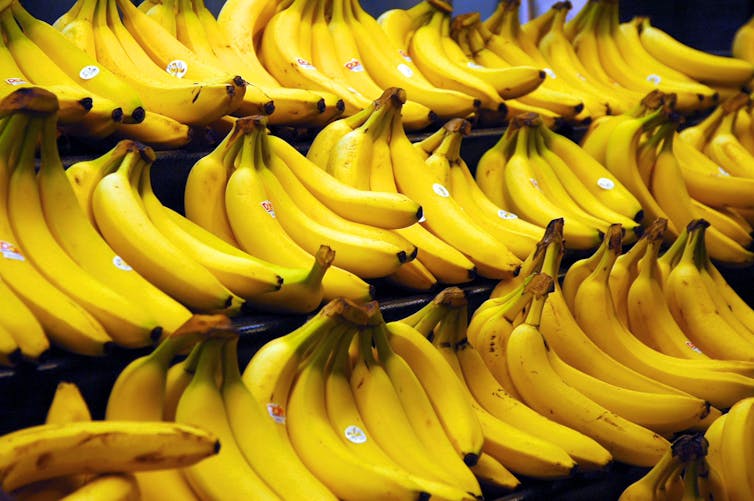
Stuart Thompson , University of Westminster
Panama disease, an infection that ravages banana plants, has been sweeping across Asia, Australia, the Middle East and Africa . The impact has been devastating. In the Philippines alone, losses have totalled US$400m . And the disease threatens not only the livelihoods of everyone in this US$44 billion industry but also the 400m people in developing countries who depend on bananas for a substantial proportion of their calorie intake.
We don’t run ads or share your data. If you value independent content and real privacy, support us by sharing.
However, there may be hope. In an attempt to save the banana and the industry that produces it, scientists are in a race to create a new plant resistant to Panama disease. But perhaps this crisis is a warning that we are growing our food in an unsustainable way and we will need to look to more radical changes for a permanent solution.
To understand how we got here, we need to take a look back at the history of the banana, and in particular the middle of the last century, when a crisis that had been growing for decades was threatening to bring down whole economies and leave thousands destitute. The banana was dying out.
A condition known as Fusarium wilt or Panama disease was wiping out whole plantations in the world’s major banana-producing countries of Latin America. It threatened an industry so important to this part of the world that some states had became known as banana republics because they were virtually governed by the corporations that produced the crop.
Because bananas of the same type are virtually genetically identical, if one plant becomes infected, all of the other trees in a plantation are also susceptible . This meant it was only too easy for Panama disease to sweep through huge expanses of vulnerable host plants. In many areas, all of the trees were killed.
Without a cure or treatment, there was no way back for a plantation once the disease had taken hold. For a while , the banana companies carved new plantations from untouched rainforests. But this act of environmental vandalism only postponed the inevitable. Soon these areas, too, became contaminated and cultivation became unsustainable. Estimates vary, but losses due to the Panama disease epidemic may have reached US$2.3 billion , equivalent to about US$18.2 billion today.
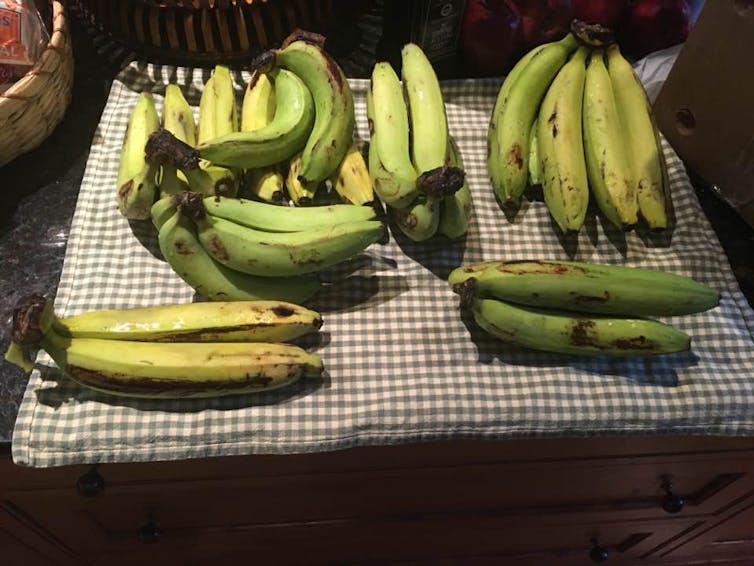
Luckily, the banana companies realised that another variety of banana known as the “Cavendish”, unlike the “Gros Michel” type grown in Latin America at the time, was almost completely resistant to Panama disease . From the 1950s, plantations of Gros Michel (or “Big Mike”) were systematically cleared and replaced with Cavendish trees.
The Cavendish had rescued the industry, and for five decades it spread further around the world. Today, 99% of exported bananas and nearly half of total production worldwide is of the Cavendish variety. But this strength has now become the banana industry’s greatest vulnerability. Panama disease has returned, and this time the Cavendish is no longer resistant.
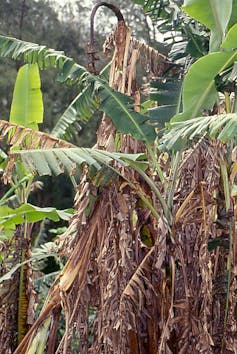
As the new strain sweeps across the world, it can only be a matter of time before this scourge returns to the huge plantations of the Caribbean and Central America. However, lessons about how to solve this latest crisis may lie in the last outbreak of Panama disease, where an answer came via an unlikely source. Not the jungles of South-East Asia, where bananas are native, but via Chatsworth House in Derbyshire , former home of the politician and keen horticulturist William Cavendish, the Sixth Duke of Devonshire.
The duke and the gardener
In 1826, Cavendish employed a young and enthusiastic farmer’s son as his head gardener. This was Joseph Paxton , who went on use the expertise he developed constructing experimental greenhouses at Chatsworth in designing the famed Crystal Palace in London.
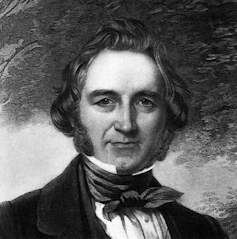
Among the exotic specimens Paxton gathered for the duke was a short banana plant he purchased for £10 from the Dorking collection of the late brewer Robert Barclay, who in turn had received it from the botanical garden in Pamplemousses on Mauritius . Paxton propagated and tended the plant for three years until it eventually produced fruit for Lord Cavendish and his guests to enjoy.
Paxton’s success with the plant, which he named Musa Cavendishii after his patron , won him the Silver Medal at the 1835 Royal Horticultural Society show. Following this fame, the nurserymen who had sold off Barclay’s collection tried to claim that the invoice for the plant should have been for £100 instead of £10. Paxton did not pay the difference.
Then began the spread of the Cavendish around the world. Bananas have a long history of migration. Archaeological evidence suggests they were first cultivated in South-East Asia and New Guinea at least 6,800 years ago, and had spread to Sri Lanka by 6,000 years ago and Uganda by 5,250 years ago. After Europeans began crossing the Atlantic at the end of the 15th century, the banana quickly followed , spreading across the Caribbean and tropical parts of the Americas.
But the 18th century Age of Enlightenment started an important new phase of propagation of varieties of banana collected on the scientific voyages of the era by amateur and professional botanists and gardeners. Many initially reached new territories because they were shared between enthusiasts who planted them in botanical or private gardens, just as Paxton did.
He and and his successors continued the trend, giving many specimens from Chatsworth to collectors and philanthropists and helping distribute the Cavendish banana around the world. They made their way to the Canary Islands, where they later came to be grown for export, probably via the gardens of a Scottish stately home and a wine merchant who immigrated to Tenerife. Specimens also reached Jamaica, where they were planted in Bath Gardens in St Thomas in 1884 .
John Williams, a missionary to the Pacific Islands was given Cavendish plants to provide food in the areas of his ministry. These specimens were initially established in Samoa in 1838, and from there the plant spread to Tonga, Fiji, Tahiti, Hawaii and Australia, as well as the original home of the banana, New Guinea. Williams did not see this himself as he was eaten in the New Hebrides in 1839 by islanders who were presumably unenthusiastic about his message.
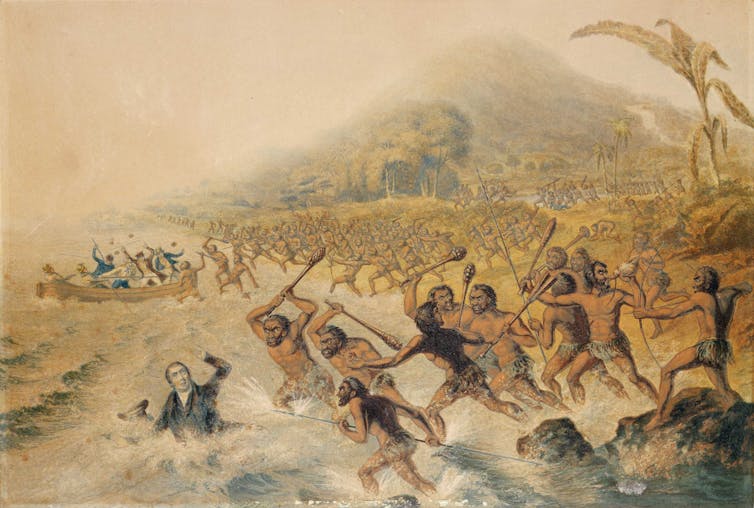
Meanwhile, the Gros Michel variety was taken from Myanmar to the St Pierre botanical garden in Martinique in the early 19th century by the French cartographer and privateer Nicolas Baudin. From there it was taken to Jamaica in 1835 by the botanist Jean François Pouyat. And the plants used to establish the banana export industry in the early 20th century probably came from these specimens.
Fortunate malfunctions
What’s surprising about the distribution of these banana plants to every part of the world hot enough for them to grow is that they’re sterile. Wild bananas are filled with large hard seeds , which makes them hard to eat. Modern bananas can’t even grow seeds. But far from hindering their spread, this genetic quirk is what has made bananas such a desirable crop. And what has left them so vulnerable.
Modern banana and plantain plants are what is known as “triploid” , meaning they have three copies of each of the chromosomes that carry their genes. As such, they cannot reproduce sexually because their chromosomes cannot be equally divided to create a sex cell, as happens in “diploid” organisms that have two copies of each chromosome (such as humans, most animals and many plants).
Triploids like this can arise when there is a malfunction in the process of forming sex cells in diploid organisms. Occasionally cells are produced that have two copies of each chromosome instead of one. When these fuse with a normal sex cell, the new plant has two chromosomes from one parent and one from the other, preventing it from making viable sex cells of its own. In the banana’s case, the plant still produces fruit but can’t make seeds.
On the surface this may appear to be a problem, but plants are not completely dependent on sexual reproduction. As any gardener knows, new plants can be started from cuttings , and new banana trees are usually produced from an existing plant by replanting root stalks, known as rhizomes, or shoots called suckers that grow out of them .
The prehistoric peoples who domesticated bananas and plantains cannot have known anything about chromosome numbers. But almost all of the many varieties that they grew are triploid. So they must have learned to look out for these fortunate accidents and cultivate them, preferring them to their wild and seedy relations.
This has important consequences, both good and bad. Plants from cuttings are clones of one another and, give or take the odd mutation, are genetically identical. This removes variety and chance from the equation. Obviously we only plant copies of trees that are vigorous and produce fruit that we like, and all of the new trees will be pretty much the same as the one we took cuttings from.
This is great for production on an industrial scale because the fruit are consistent and if you harvest and treat them in the same way, they will all be ripe and ready to eat at the same time . Unfortunately it is also great for any disease that infects them because if it gets a foothold in one tree, those nearby will also be vulnerable , as will their neighbours, and it can spread through the whole plantation. Which is exactly what is happening now.
Panama returns
The earliest known discovery of Panama disease was actually in Australia in 1874. First the leaves of the banana trees stopped growing. Then they started to curl and wilt. Eventually the trees dried out completely and died. In 1890, the disease was found in its namesake country and over the next 30 years spread to most Caribbean and Central American countries.
It took a while to identify the cause, but in 1910, it was found to be the wilt fungus Fusarium oxysporum cubense or “Foc” for short. The plants died because the channels that carry water and minerals from the roots to the leaves became blocked. It was initially thought that these conduits became clogged by the fungus but we now know that the plant itself plugs them , presumably in a vain attempt to stop the fungus spreading.
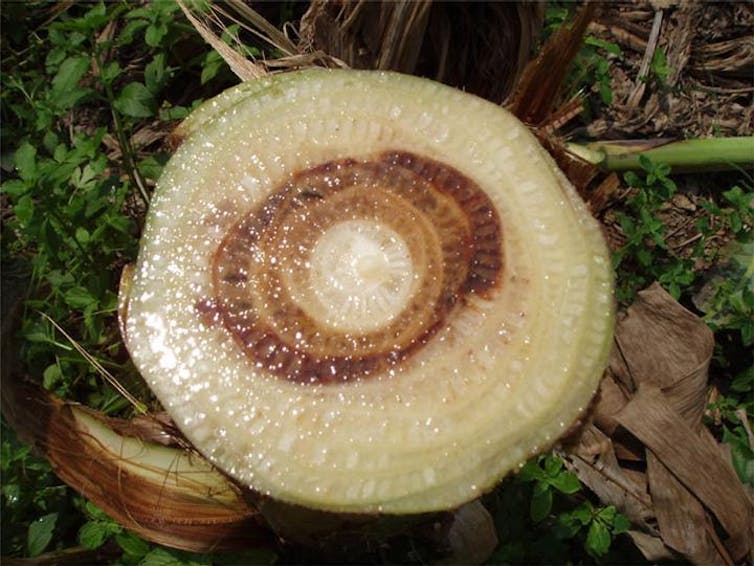
We also now know that Foc is spread by contaminated soil . A minute amount of tainted soil can carry the disease to a new plantation where it remains infectious for decades , immune to any chemical treatments. This was the situation facing the banana industry in the 1950s, when Gros Michel plantations around the world were being overwhelmed.
Cavendish bananas require more protection than Gros Michel, and were thought of as small and flavourless in comparison. But given the Cavendish’s apparent immunity to Foc, and otherwise facing total collapse, the industry had no alternative but to switch.
For a while, it looked as if the banana had been saved. Then, in the late 1960s, another outbreak of Panama disease was discovered in Taiwan, this time in a plantation of Cavendish plants. By the early 2000s, only 6,000 hectares of banana plantations out of a former 50,000 hectares in Taiwan remained.
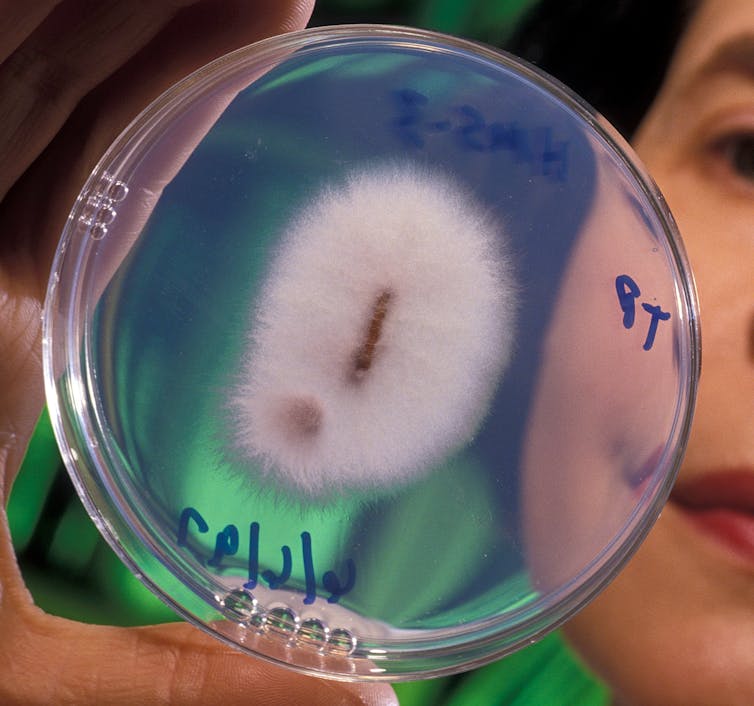
This puzzling development was down to the fact that, just as there are different varieties of bananas, there are different types of Foc. The Gros Michel trees were infected by what is known as “Race 1” . The strain of fungus that appeared in Taiwain is known as “Tropical Race 4” or TR4 . It can infect not just Gros Michel, but also Cavendish bananas and as many as 80% of the varieties in cultivation. (Although this assumes that plantains are also susceptible and so far there is little conclusive evidence either way.) So now the industry is once again facing disaster, what can be done to save it this time?
A genetic solution?
The simplest response is quarantine. Panama disease was so devastating in the 20th century because effective measures to control its spread came too late. It is also possible that the first TR4 outbreak in Taiwan could have been nipped in the bud if the magnitude of the problem had been recognised earlier . But it seems that the innate resistance of Cavendish plants to Race 1 encouraged complacency until the epidemic was out of control.
Can we stop the spread by preventing infected plant material and soil reaching new areas? Unfortunately, it’s not necessarily that easy . Foc can lurk in minute patches of mud on a wheel or shoe. People, machinery and everything else coming into a plantation have to be stringently controlled. Imagine operating what is essentially a farm with all non-essential people and vehicles excluded and with changing and decontamination zones for those you have to let in.
It worked for a while in Australia, which has very strict rules to prevent foreign soil entering the country, but even there the defences were breached in 2015 . There are always slip ups, and people who ignore the rules. In many areas there are unprotected banana plants growing wild or in villages and if these become infected they can act as bridges for the disease to cross from one plantation to the next. Quarantine may slow the march of TR4, but in the long term we really need a banana that is resistant to the fungus.
Here, the triploid nature of the banana presents an unwanted complication. Historically, new crop varieties were bred by crossing plants with desired characteristics until they were combined in a single new variety. For example, cross breeding a plant that produces a good yield for farmers with another that was disease resistant. But crossing domesticated bananas doesn’t produce any seed and so this is not usually an option.
However, genetic modification offers other ways to move properties between plants (and other organisms). In principle this could offer a solution and there are already some promising results. Researchers in Australia have found that adding two different genes to the genetic code of Cavendish bananas protects the plants from TR4. The first was taken from a wild banana resistant to TR4 and is one of a large family of genes that recognise invading diseases so that the plants can protect themselves.
The second comes from a more unlikely source: nematode worms . There are occasions when organisms need some cells to sacrifice themselves . A dramatic example is when a tree sheds its leaves for winter but it happens in our own development too. In the womb, your fingers are formed from flipper like appendages when the cells that separate them die off . The nematode gene is one that blocks this process.
This seems a strange way to protect the plant but it might be effective against TR4 in bananas because, as we saw earlier, the invading fungus may actually hijack this process , using chemical messages to programme the banana cells to self destruct. The nematode gene may work by blocking these signals .
Using these methods, we could carry on eating the bananas that we are used to or even see if the same would work to help bring back the tastier Gros Michel. But these resistant Cavendish bananas are now GM crops. People in many countries have become used to eating GM food , but not so in Europe, which has the most stringent GM regulations in the world. Perhaps European regulators could be persuaded to make an exception if it was a case of GM bananas or none at all. But we might need to look for another solution.
Chromosome maths
One alternative could be to make new triploid seedless plants from scratch. Picking out the occasional tree that produced seedless fruit must have been a slow process when bananas were first domesticated. But now that we understand the process, we can make our own triploid plants much more easily.
This is usually done using plants with four copies of each type of chromosome called tetraploids. These often grow faster and produce sturdier plants that can withstand stress better than their diploid relations (many of our crops have expanded chromosome numbers). But they are especially useful in plant breeding because they produce sex cells with two copies of each chromosome. This allows the creation of otherwise impossible infertile hybrids.
When you cross a tetraploid (with two copies of every chromosome in each sex cell) with a normal diploid plant (with only one copy of each chromosome per sex cell) you get a triploid. A triploid plant can’t produce its own sex cells. So if it is a banana, its fruit will be seedless.
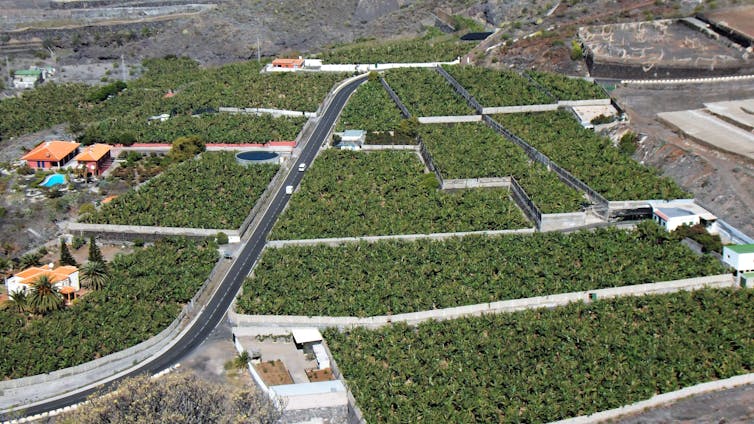
The same result can be achieved by taking an infertile cross between species, the plant equivalent of a mule, and exposing it to chemicals that cause it to double its chromosomes and become tetraploid. A hardy hybrid of wheat and rye called triticale was made in this way .
Some breeding programmes have made tetraploid plants by crossing triploid and diploid varieties , but this depends on infrequent genetic events and so it takes time and effort. A quicker way is to force chromosomes to double using a chemical called colchicine.
From these approaches we now have a number of tetraploid synthetic banana hybrids and some have proved to be resistant to TR4 . These plants are not very useful commercially because they are fertile so produce seed-filled bananas. But they can be crossed with one another to bring together useful traits, and then with ordinary diploid trees to make a new generation of triploid seedless bananas. This approach has also created some new hybrids with resistance to TR4 , but none so far with the flavour and consistency that we want in a replacement for Cavendish.
A slightly more radical approach is to try to evolve plants resistant to TR4. Cavendish plants are clones but their genetic code can become slightly different over time because of mutations and changes in the way their DNA is read.
One group in Taiwan has been exposing Cavendish banana seedlings to soil contaminated with TR4 and looking for those that survive a little bit better than the others. They pick these out and use them for their next trial. Only two or three plants of every 10,000 show promise but after many iterations they now have a Cavendish line with some ability to withstand TR4 .
However, none of these potential solutions deal with the fact that farming huge plantations of cloned trees is an intrinsically unstable way of doing things. They may offer economies of scale, keeping prices down and offering consistent tasty fruit. But, even with a solution to TR4, how long will it be before the next disease takes hold?
Perhaps we should be using more varieties of bananas and growing them alongside other crops or in alternation with them. That way, an infection won’t find such huge swathes of susceptible hosts close together, as can happen now.
There is also evidence that some other crops can protect bananas against TR4. One study found that bananas planted in TR4-contaminated soils largely escaped infection after being grown in the same fields as Chinese leeks for three years. This seems to be because Chinese leeks release chemicals that kill fungi. Cassava also clears fields of TR4 , perhaps because of antifungal substances produced by the cassava itself and by microorganisms associated with its roots .
The Cavendish banana may have had a remarkable journey from colonial curiosity to global staple. But its success has helped create a food system with a fatal flaw (though this problem of monoculture farming is hardly unique to bananas ). Perhaps we ultimately need to be prepared to accept less standardised and slightly more expensive food in order to accommodate less intense and more diverse agriculture. You might never be quite sure what to expect when you peeled a banana, but the result could be a more robust and sustainable food system without a major crisis every few decades.
Stuart Thompson , Senior Lecturer in Plant Biochemistry, University of Westminster
This article is republished from The Conversation under a Creative Commons license.